Cell EMF Calculator – Electromotive Force of a Cell
Cell EMF calculator is a simple tool that provides you with the value of electromotive force (EMF) for any galvanic (voltaic) cell with a given electrode potential. If you are a bit confused with all these terms, don't worry!
In the article below, you will find a brief description of what EMF is, how to calculate EMF, and examples of electromotive force sources. Moreover, we prepared an explanation of a redox reaction and a galvanic cell. A short step-by-step tutorial demonstrates the usage of our cell EMF calculator. Scroll down to keep reading!
What is EMF?
EMF is an abbreviation of the electromotive force (see Faraday's law calculator). By definition, EMF is a force or an electric pressure that generates a current in a circuit. The EMF units are volts [V].
To understand this definition better, consider a typical galvanic or voltaic cell consisting of two electrodes. The electromotive force is the potential difference between them (see the Ohm's law calculator).
Additionally, the electromotive force of a galvanic cell specifies the ability of an electrochemical cell to cause the flow of electrons through the electric circuit.
EMF equation
The electromotive force of the electrochemical cell can be calculated using the equation:
EMFcell [V] = Ecathode [V] - Eanode [V]
where Ecathode is the potential of the cathode (in volts) and Eanode is the potential of the anode (in volts). Remember that in a cell, the potential of the cathode is higher than the potential of the anode.
Electropotential of anode and cathode
If you want to find the potential of a metal electrode, one way is to check the galvanic series and find the particular metal in the table. The second way is to calculate it using the Nernst equation, also known as the cell potential equation. This formula allows you to calculate the reduction potential of a half-cell or full-cell reaction. Check how to do it with our Nernst equation calculator.
In the table below, you can find electro potential of metals:
Electrode | E⁰ [V] |
---|---|
Li / Li⁺ | -3.04 |
Ca / Ca²⁺ | -2.86 |
Mg / Mg²⁺ | -2.36 |
Al / Al³⁺ | -1.69 |
Mn / Mn²⁺ | -1.18 |
Zn / Zn²⁺ | -0.76 |
Cr / Cr³⁺ | -0.74 |
Fe / Fe²⁺ | -0.44 |
Cd / Cd²⁺ | -0.40 |
Co / Co²⁺ | -0.28 |
Ni / Ni²⁺ | -0.26 |
Sn / Sn²⁺ | -0.14 |
Pb / Pb²⁺ | -0.14 |
Fe / Fe³⁺ | -0.04 |
H₂ / 2H⁺ | 0.00 |
Bi / Bi³⁺ | +0.32 |
Cu / Cu²⁺ | +0.34 |
Ag / Ag⁺ | +0.80 |
Hg / Hg²⁺ | +0.85 |
Au / Au³⁺ | +1.52 |
How to calculate EMF?
Take a look at the EMF calculation example below.
Let's consider a Daniell cell – a simple electrochemical cell invented in 1836 by John Frederic Daniell (British chemist and meteorologist). In this cell, copper is immersed in a solution of copper(II) sulfate, and zinc is immersed in a solution of zinc sulfate.
Cell's schema: (−) Zn | Zn2+ || Cu2+ | Cu (+)
-
Check the metal's potential: the electrode potential of zinc is -0.76 V, and copper is +0.34 V ⇒ because of the lower potential, zinc is the anode, and copper is the cathode. Type these values into our cell EMF calculator.
-
Calculate the EMF value of the electrochemical cell using the equation:
EMFcell = +0.34 V - (-0.76 V) = 1.10 V
Here it is! The electromotive force of the Daniel cell is equal to 1.10 volts.
Electromotive force sources
Below you can find some examples of EMF sources:
- Batteries;
- Alternators;
- Galvanic cells;
- Solar cells;
- Fuel cells;
- Thermocouples; and
- Some living organisms (e.g., electric eel).
Each of the EMF sources listed above contains an energy source that induces a flow of electric charges. These sources may be chemical processes (in batteries, fuel, and galvanic cells), mechanical forces (in alternators), electromagnetic radiation emitted by Sun (in solar cells), and temperature differences (in thermocouples).
Electrochemical cell types
An electrochemical cell is one of the types of energy source. It can be created by placing metallic electrodes in an electrolyte where a chemical reaction either generates or uses an electric current. Electrochemical cells which generate an electric current are called voltaic cells (named after their inventor, the Italian physicist Alessandro Volta) or galvanic cells (named after the Italian physicist and physician Luigi Galvani). Common batteries consist of one or more such cells. In other electrochemical cells, an externally supplied electric current is used to drive a chemical reaction (which would not occur spontaneously).
Galvanic cell
In any electrochemical process, electrons (gained by a redox reaction) flow from one chemical substance to another. Two metal electrodes (anode and cathode) immersed in a salt solution create a galvanic cell, which induces the flow of an electric current. These metals must have a different tendency to lose electrons. The cathode (+) is an electrode with higher electric potential, and the anode (-) is the one with lower electric potential.
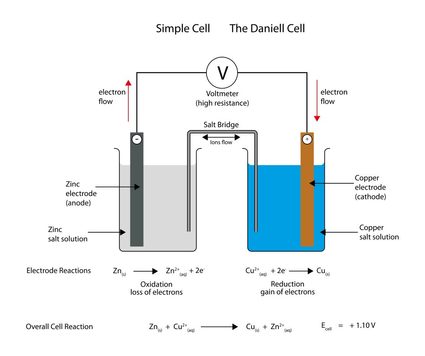
The oxidation half-reaction occurs at the anode, and the reduction half-reaction occurs at the cathode. Both the anode and cathode are electrodes, but the anode is negatively charged, while the cathode is positively charged. The conventional current flow is from the cathode to the anode, while electrons flow from the anode to the cathode.
Redox reaction
Reduction and oxidation processes only occur together (it is not possible that these reactions will happen separately). That is why the redox reactions can be described as two half-reactions, one representing the oxidation process and one the reduction process. Let's see it in the example of the Daniell cell. In the Daniell cell, copper is immersed in a solution of copper(II) sulfate, and zinc is immersed in a solution of zinc sulfate. In this cell, zinc acts as the anode (because of lower electric potential), and copper acts as the cathode (because of higher electro potential):
- E⁰ of zinc electrode = -0.76 V
- E⁰ of cooper electrode = +0.34 V
Overall Daniell's cell chemical reaction: Zn(s) + Cu²⁺(aq) → Zn²⁺(aq) + Cu(s)
Oxidation (on anode): Zn(s) → Zn²⁺(aq) + 2e⁻
Reduction (on cathode): Cu²⁺(aq) + 2e⁻ → Cu(s)
The number of electrons lost by the reductant is equal to the number of electrons received by the oxidant (for any redox reaction).